The Accelerating Universe
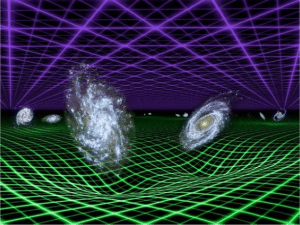
A visualization of the attractive force of gravity (green grid) and the repulsive force of dark energy (purple grid). From dailygalaxy.com.
In the early 20th century, Albert Einstein famously introduced the idea that gravity causes the curvature of space and time in his theory of general relativity. Included in this theory was a term called the Cosmological Constant, a repulsive force to explain why the universe didn't collapse in on itself under the weight of its own gravity.
Upon learning from Edwin Hubble that the universe was not in stasis, as he had assumed, but was in fact expanding, he removed this idea from his theory. But in 1998, this idea was re-introduced by two teams of researchers who discovered that the universe was not only expanding - it was accelerating.
The reason for this cosmic acceleration remains both a mystery and the impetus behind my research. Is the force behind this acceleration, the so-called "dark energy", a fifth force similar to gravity or electromagnetism? Is it a property of the vacuum of space? Or do we understand less about the laws of gravity than we thought we did?
My research measures the expansion of the cosmos starting with the local universe and continuing out beyond 10 billion light years. By measuring the speed at which the universe expands at different epochs across cosmic time, we hope to deduce the reason behind the expansion - the nature of dark energy.
A Cosmic Beacon
To measure cosmic expansion, we need to know the distance to galaxies and the speed at which they move away from us. The most direct method is to use what we call a "standard candle": an astrophysical object or event of known intrinsic brightness, so that when we observe how bright it appears, it is possible to deduce the distance.
The most luminous of these standard candles are called Type Ia supernovae. Type Ia supernovae occur when a burned-out star (called a white dwarf) that was once about the size of our sun gains matter from a second, nearby star until it reaches a point at which it begins to collapse under its own gravity. During the collapse, thermonuclear fusion starts a runaway chain reaction that detonates the white dwarf in a massive explosion that can be seen throughout most of the observable universe.
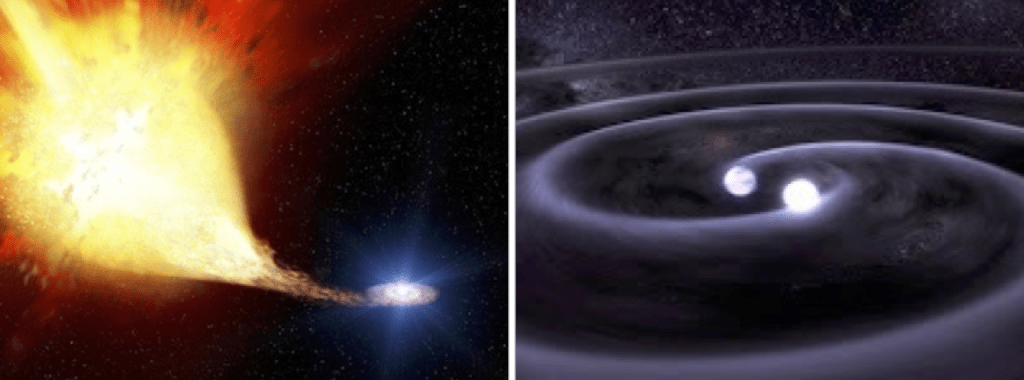
Two scenarios for how a Type Ia supernova might form - with a second star pouring mass onto a white dwarf (left) or two white dwarfs merging together (right). © ESA and Justyn Maund (Queens Univ. Belfast) (left). © NASA, Tod Strohmayer (GSFC), and Dana Berry (Chandra X-ray Observatory) (right).
It is thought that because the mass at which these white dwarfs explode is uniform, they shine at nearly the same intrinsic brightness every time one detonates. Although they are quite rare, I work with dedicated supernova surveys such as CANDELS and CLASH (with the Hubble Space Telescope), and Pan-STARRS, which observe tens of thousands of galaxies, waiting for a supernova so that we can measure its distance and velocity (by measuring how red the light appears due to the Doppler effect).
The Pan-STARRS Telescopes
In the last few years, we learned more about dark energy than ever before thanks to the Pan-STARRS telescope - a supernova discovery machine. Pan-STARRS used a 1.4 gigapixel camera to discover over 5,000 supernovae within four years. I recently used approximately 1,200 of those supernovae to measure the dark energy equation-of-state parameter, the ratio of pressure to density of dark energy. This measurement is a clue to the nature of dark energy and helps to distinguish a cosmological constant
model from alternative models. With my collaborators (led by Dan Scolnic), I also worked to combine Pan-STARRS supernovae with those from other surveys and measure dark energy better than ever before.
In the last year, as Project Scientist of the Young Supernova Experiment, we've been using Pan-STARRS in the more local universe to learn even more about dark energy. YSE is a supernova discovery engine that has observed more than 1,000 SNe to date and has observed about 8% of the supernovae discovered so far this year. With thousands of supernovae set to be discovered by YSE, we will not only lower the uncertainties on the properties of dark energy, but we can also test the laws of gravity better than ever before.
The Hubble Constant
The best end-to-end test of our cosmological model is the measurement of the Hubble constant - the speed at which the universe is currently expanding. We begin with observations of the cosmic microwave background, light emitted just 380,000 years after the big bang. The imprint of sound waves in the cosmic microwave background can still be seen today, and the size of the sound horizon
combined with our best model of dark matter, dark energy and the physics of the universe gives a prediction for how fast the universe should be expanding today.
Using the cosmic distance ladder, we can also directly measure how fast the universe is expanding. First, we measure the distance to nearby variable stars through parallax - a technique that measures geometric distances from the apparent shift of stars relative to background stars as the Earth moves around the sun. The intrinsic brightness of those variable stars is the same as the intrinsic brightness of those same variable stars seen in other galaxies. And if a Type Ia supernova has occurred in those galaxies, we may then deduce the intrinsic brightness of that supernova. Type Ia supernovae can be seen far enough away that they are part of the Hubble flow
- the overall expansion of the universe, not affected by gravitational interactions between nearby galaxies. From the velocity and distance to those Type Ia supernovae, we measure the Hubble constant.
Currently, we are failing this test of our cosmological model. We are 99.98% confident that the predicted Hubble constant from the cosmic microwave background does not agree with what we actually measure. That means new physics could be in play.
I work with the Young Supernova Experiment (and its predecessor, the Foundation Supernova Survey), new surveys on the Pan-STARRS telescopes that measure distances of nearby Type Ia supernovae better than every before. I work to understand whether distances measured using Type Ia supernova are affected by whether those supernovae exploded in star-forming galaxies. The type of galaxy in which a supernova forms determines some of the properties of its progenitor star, and could change the distance we measure. So far, we find some interesting new hints of how supernova distance measurements change from galaxy to galaxy, but that the measurement of the Hubble constant is minimally affected.
Rubin and Roman: the Future of Dark Energy
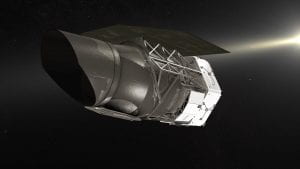
An artist's conception of WFIRST
We don't have to look far into the future for a glimpse of how our understanding of the physics of the universe may soon be transformed. The Vera C. Rubin Observatory will begin survey operations in 2022, discovering millions of supernovae and creating 15 terabytes of data per night . The Nancy Grace Roman Space Telescope, a new space telescope with a planned launch in the mid-2020s, is designed to revolutionize our understanding of dark energy. As part of the LSST Dark Energy Science Collaboration and a Roman Science Investigation Team, I am working to help build these future supernova surveys.